* Professor Mori, co-spokesperson of the MEG collaboration, announced the experiment's latest research findings at the La Thuile 2016 international conference in Italy.
Professor Toshinori Mori (ICEPP, The University of Tokyo)
Associate Professor Wataru Ootani (ICEPP, The University of Tokyo)
Professor Satoshi Mihara (Institute of Particle and Nuclear Studies, KEK)
Key points
- Muon to electron + gamma decay (μ→eγ) was studied for four years with unprecedented sensitivity. Despite numerous theoretical predictions, μ→eγ decay was not discovered. This places stringent limits on new physics models explaining the origin of neutrino oscillation and grand unification.
- The MEG collaboration performed the experiment using innovative detectors newly developed by the University of Tokyo and KEK research teams and high-quality and the world's highest intensity muon beam provided from the accelerator at the Paul Scherrer Institute (PSI) in Switzerland. This experiment's sensitivity was roughly 30 times higher than that of previous experiments.
- While MEG has ended, the experience gained through the experiment is being used to prepare an upgraded version of the experiment, named MEG II. The collaboration plans to launch MEG II next year, further increasing sensitivity 10-fold.
Contents of announcement
Research background
A great deal of research has been performed on grand unified theories as new elementary particle theories that go beyond the Standard Model. In the 1980s, Distinguished Professor Masatoshi Koshiba began the Kamiokande experiment to attempt to observe proton decay and to verify grand unified theories. According to the grand unified theories, immediately after the Universe was born, all the interactions between elementary particles were unified. The breakdown of this unified force resulted in cosmic inflation, creating our Universe today. In the 1990s, an experiment at the European Organization for Nuclear Research (CERN) conducted by an international collaboration including the University of Tokyo suggested an extended version of grand unified theory that involved supersymmetry. This became the most promising candidate for new physics beyond the Standard Model. However, the new theory is unable to be fully tested even with Super-Kamiokande. In the late 1990s, Riccardo Barbieri et. al. proposed that the new grand unified theory predicts the occurrence of μ→eγ decay, which was prohibited in the Standard Model.
On the other hand, the phenomenon of neutrino oscillation, discovered through the Super-Kamiokande experiment in 1998, demonstrated that neutrinos have mass. The masses of neutrinos are far less than those of other elementary particles, which suggests that the mechanism by which neutrinos get mass differs from that of others. This mechanism is called the seesaw mechanism, proposed by Murray Gell-Mann, Tsutomu Yanagida et. al. According to the seesaw mechanism, there may have been particles like extremely massive neutrinos in the early Universe, and their decay may have eliminated the anti-particles, leaving only particles. Junji Hisano and others pointed out that the massive neutrino-like particles induce μ→eγ decay.
The grand unified theories and the seesaw mechanism predict μ→eγ decay, but the probability of this decay is roughly one in one trillion. There had been no prior experiments that measured particle decay with such a low probability, and it was believed impossible using existing particle detectors.
Items this research seeks to elucidate
As stated above, the grand unified theories and seesaw mechanism, which is believed to be the source of neutrino oscillation, predict that μ→eγ decay will occur with a probability of one in one trillion. This decay is prohibited in the Standard Model. In this research, new, high-performance particle detectors were developed and an experiment was performed to discover proof of the grand unified theories and seesaw mechanism by detecting this one-in-a-trillion μ→eγ decay. If μ→eγ decay were detected, the decay probability and decay angular distribution could be used to investigate the properties of the new physics. If this decay were not detected, it would prompt a major revise of new physics scenarios that describe the origin of the Universe.
New measurement technologies developed for this research
It would not be possible to detect particle decays with a probability of one in a trillion using existing detectors. The University of Tokyo, KEK, and Waseda University therefore engaged in joint research to develop two devices: a 2.7 ton liquid xenon detector, the largest in the world, capable of measuring gamma rays with an unprecedented level of accuracy, and a special superconductive spectrometer, capable of rapidly processing tremendous amounts of decaying particles. The only accelerator capable of generating almost 100 million muons per second, necessary for this research, was located at the Paul Scherrer Institute (PSI) in Switzerland. Therefore, the Japanese research group, led by the University of Tokyo, submitted its experimental proposal to PSI. With PSI's approval, the team was joined by research groups from Switzerland, Italy, Russia, and the U.S., conducting research through the MEG international joint experiment. After a pilot experiment in 2008, the experiment intermittently acquired data over a roughly four-year period, from late 2009 to mid-2013.
Research results and spill-over effects
The research team successfully analysed the data collected over the four-year period to search for evidence of μ→eγ decay with an unprecedented sensitivity (capable of identifying decay even at incidences as low as one in two trillion). Unfortunately, despite theoretical predictions, even with this search sensitivity the researchers were unable to observe μ→eγ decay. This indicates that μ→eγ decay occurs with a probability of less than one in 2.4 trillion. The results conflict with the simple grand unified theories and seesaw mechanism scenarios previously envisioned. This places new, stringent limits on the potential of new theories that go beyond the Standard Model in describing the birth of the Universe.
CERN's LHC accelerator increased the collision energy last year and the experiments were resumed, but again failed to discover new particles that were not described by the Standard Model. While LHC experiments primarily search for new particles that interact with quarks and gluons, μ→eγ decay is highly sensitive to new particles that interact with electrons and neutrinos, so the research is mutually complementary and synergistic. Combining results from LHC experiments and MEG experiment results in an even harsher outlook for the supersymmetric grand unified theory, and around the world a great deal of thought is being dedicated to developing a new theoretical framework.
Future plans
Preparations are underway for MEG II, an upgraded experiment that leverages the experience gained through MEG (Fig. 1). The MEG II experiment will use several newly developed measurement technologies that did not exist when MEG was designed. It is expected to reach ten times the sensitivity of MEG and aims to detect μ→eγ decay with incidences as low as one in 25 trillion. The new measurement equipment is currently being constructed, and is expected to be completed this year, followed by its commissioning. If everything progresses smoothly, experimentation will begin in 2017, and it will take at least three years of data collection to reach its ultimate sensitivity. It will compete with and collaborate with LHC experiments to continue research that explores the origins of neutrino oscillation and grand unified theory.
● Contents of simultaneous release by PSI: Related site
This research was supported by JSPS KAKENHI Grant-in-Aid for Specially Promoted Research JP22000004 "MEG Experiment ― From Lepton Flavour Violation toward Grand Unified Theory" (PI: Toshinori Mori) and JP26000004 "MEG II Experiment - Highest Sensitivity Search for Rare Muon Decay to Explore Grand Unified Theories" (PI: Toshinori Mori), as well with the assistance of the Paul Scherrer Institute (Switzerland), the National Institute for Nuclear Physics (Italy), the U.S. Department of Energy (DOE DEFG02-91ER40679), etc.
Publications
Journal name: The European Physical Journal C (vol. 76, 434). The online version is available in the e-print server http://arxiv.org/abs/1605.05081
Title of paper: "Search for the lepton flavour violating decay μ+→e+γ with the full dataset of the MEG experiment"
Authors: A. M. Baldini, et. al.., MEG Collaboration (authors' names in alphabetical order)

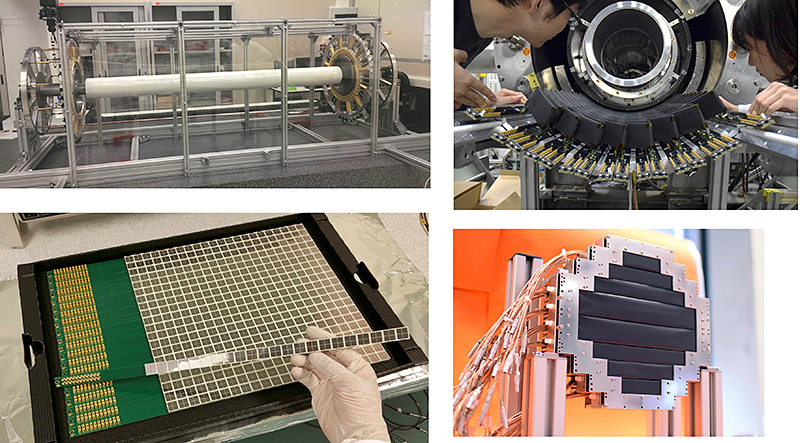
(Bottom left) Newly developed ultra-sensitive optical sensors (1/10 of entire unit) (Bottom right) Completed radiative decay counter