Tabletop Experiment
Exploring the fundamental nature of vacuum using optical technologies
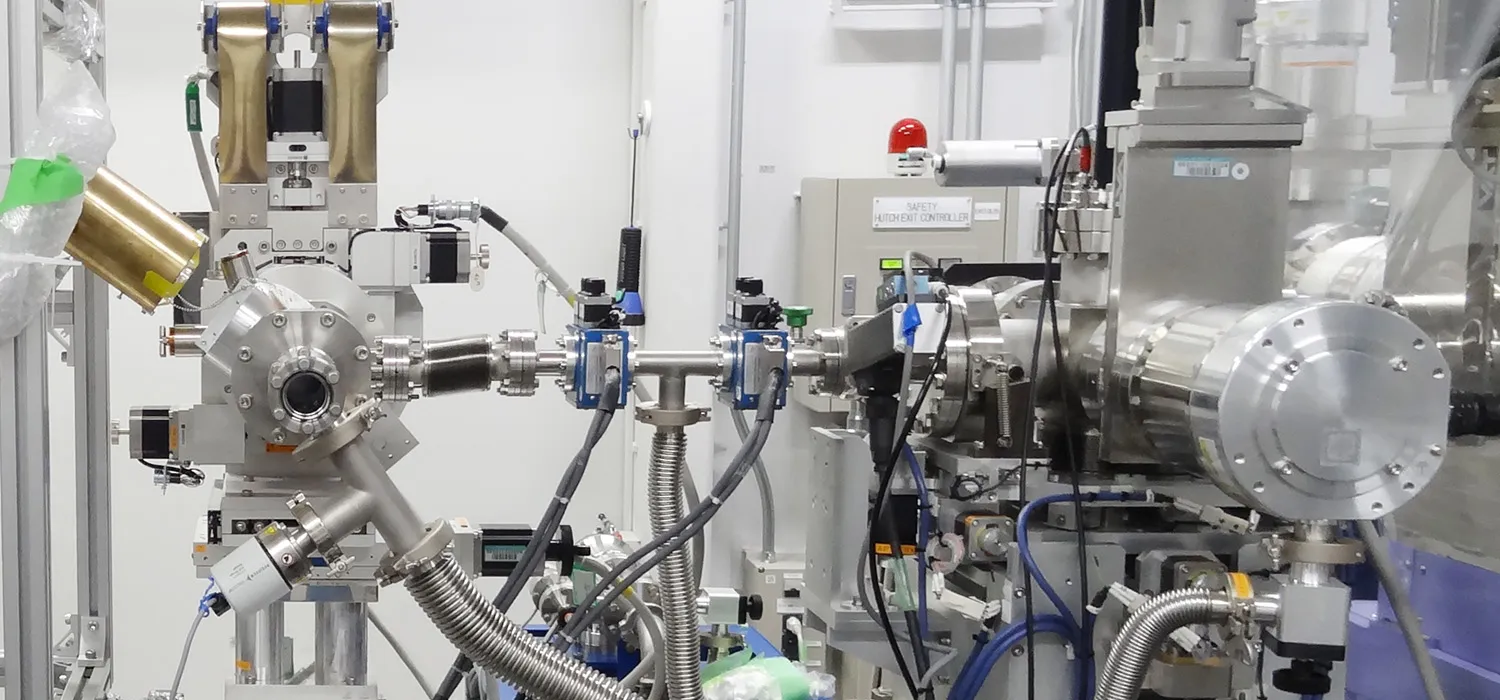
Overview
Tabletop experiments are small-scale experiments that use unique ideas and diverse experimental methods to explore unknown phenomena.
Instead of using large accelerators, these experiments use optical technologies. The suitable kinds of light for the experiments are selected from a wide range of wavelengths extending over 12 orders of magnitude, from radio waves to visible light and gamma rays. The rapid advances in quantum optics in recent years have made it possible to freely control the accuracy, intensity, and wavelengths of light. These experiments combine various optical technologies to explore the fundamental nature of vacuum.
When you hear the word "vacuum," you may imagine a completely empty space, but in modern physics, the vacuum is described as a space with complex structures in which virtual particles are continuously being generated and annihilated. When a strong magnetic field is applied to the vacuum, the vacuum is distorted by virtual particles which sense the magnetic field and become anisotropic. The goal of these experiments is to measure this vacuum distortion with optical technologies.
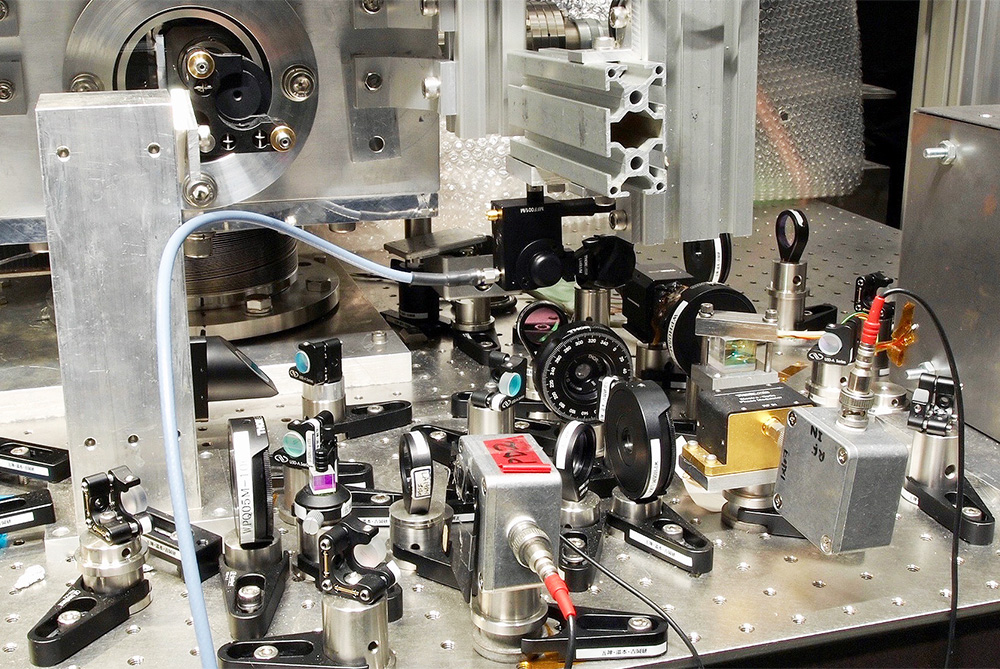
Under a strong magnetic field, the polarization of infrared light is slightly perturbed by the distortion of the vacuum. To detect this, a high accurate infrared laser is reflected between two mirrors, and the perturbation is amplified. X-rays are also suited for the observation of the tiny vacuum distortions. Because the wavelength of X-rays is roughly 1/10,000 as long as that of infrared light, the distortion can be measured with a small scale (roughly a micrometre scale) with X-rays. SPring-8 Angstrom Compact free electron LAser (SACLA), an X-ray free electron laser located in Riken, is used as a high-precision X-ray light source. There are various ways to produce the vacuum distortion, such as the collision of powerful lasers or the splitting and colliding X-rays. Each of these experiments are the first trials in the world.
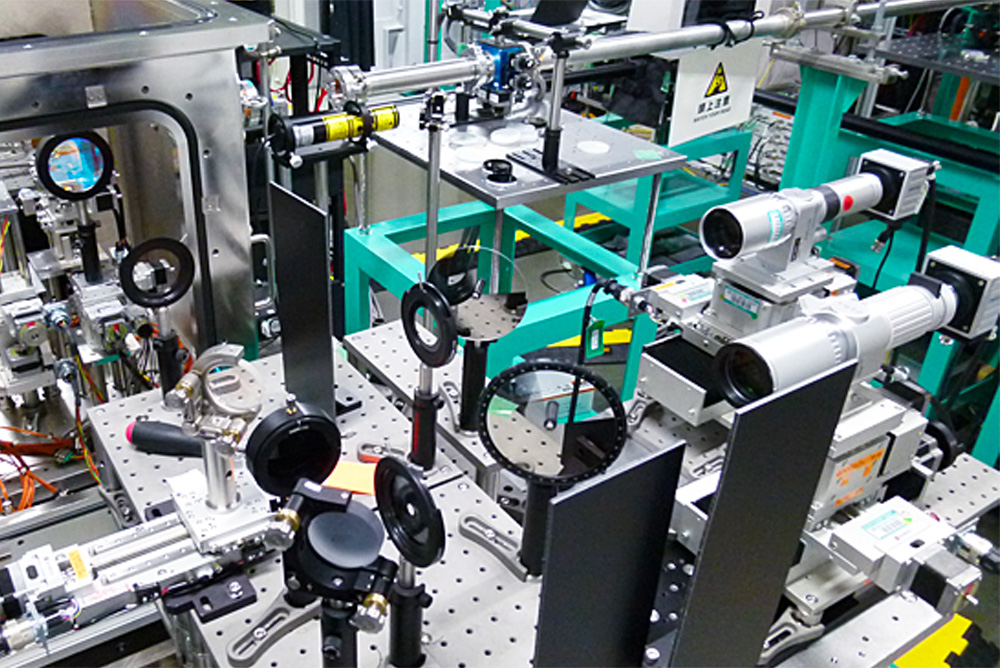
Tabletop experiments also focus on the experiments using "positronium," a quasi-stable particle consisting of an electron and its anti-particle, a positron. This structure makes positronium extremely useful for investigating the symmetry of matter and antimatter. There are two different states, para-positronium and ortho-positronium, depending on their spin states. The former has properties very similar to those of vacuum, while the latter has properties very similar to those of light. By examining the differences between the two, we can study the properties of vacuum.
So far, we have measured the energy differences between the two with a precision level of 1/1,000,000, and our results agree well with theoretical predictions. We have also jointly developed a millimetre wave high-intensity light source together with the University of Fukui, and by irradiating ortho-positronium with this light, we have observed the stimulated transition of ortho-positronium to para-positronium for the first time in the world. We are currently developing a device that will condense ortho-positroniums with lasers, enabling macroscopic observation of the transformation of ortho-positronium into para-positronium. This will further our understanding of the properties of the vacuum and make it possible to create gamma ray lasers.
We will use diverse optical technologies such as these to explore vacuums and various elementary particle phenomena.
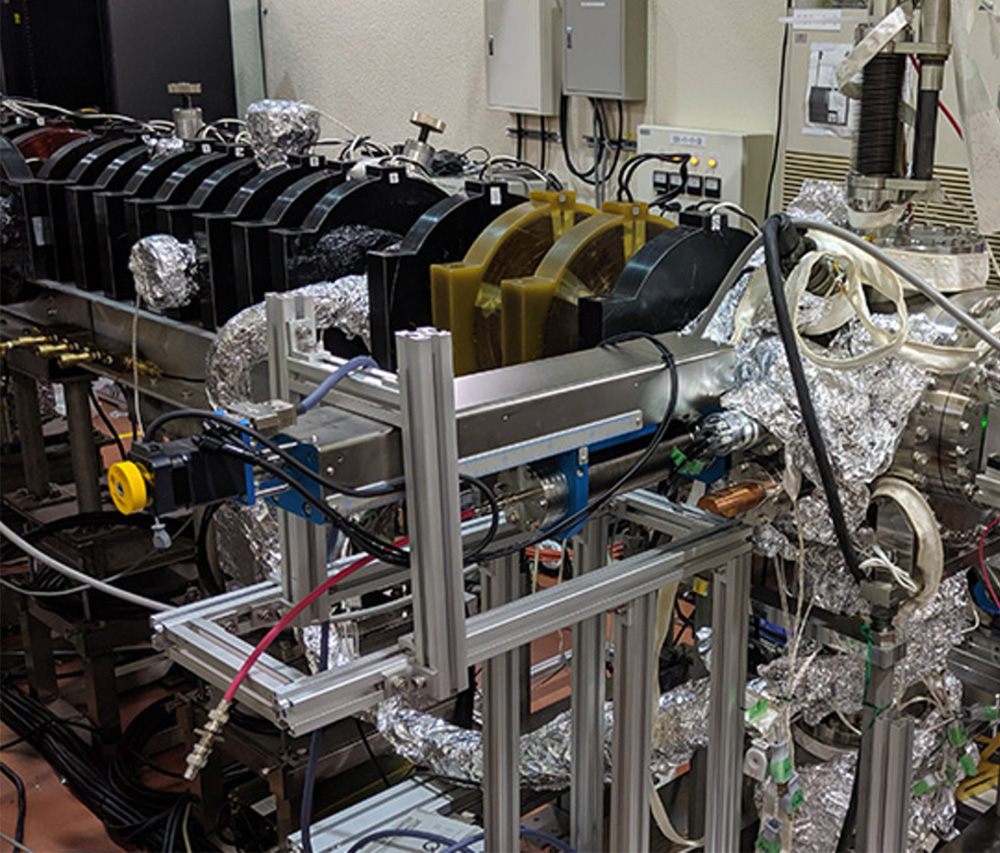
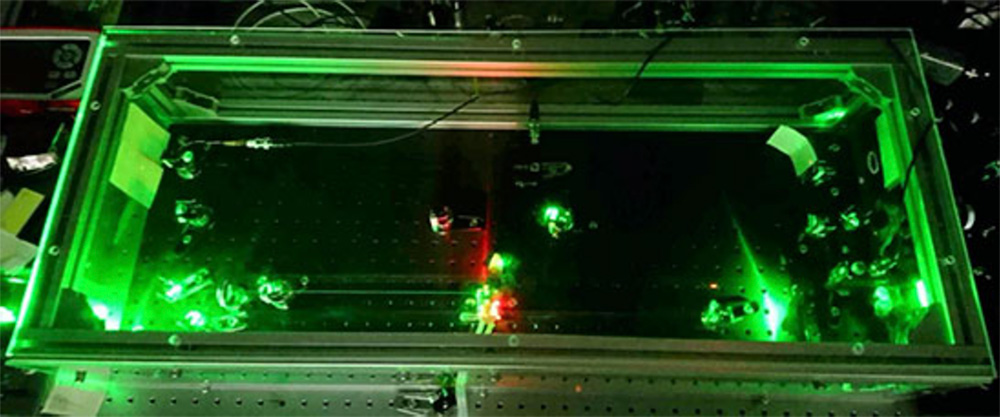
What is positronium?
Electrons, which are elementary particles, have paired anti-particles, called positrons. While electrons have negative charges, positrons have positive charges, so they are electrically attracted to each other, creating a quasi-stable particle similar to a hydrogen atom. This is a positronium. Because it consists of a particle-antiparticle pair, it has a short lifetime and decays into gamma-rays. Because of its simplicity, precise theoretical computations are possible for positronium, and thus, they are extremely useful to verify elementary particle theories. Its properties, such as the difference of the spin-dependent energy level (hyper-fine structure) and its lifetime, have been precisely calculated, and tabletop experiments are experimentally verifying these calculations.
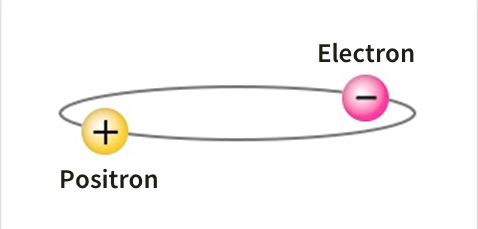
What are X-ray free electron lasers?
X-rays are light which has 100 to 100,000 times higher energy than visible light. Although X-rays are commonly used in applications such as medical X-ray imaging, their X-ray sources are like filament bulbs and not highly suited for physics experiments. X-ray free electron lasers, on the other hand, produce laser-like X-ray beams by controlling accelerated electrons. X-rays with required energies can be emitted in short pulses at high intensities. There are only two of these devices in operation around the world. One of them is SACLA, in Harima, located in Japan's Hyogo Prefecture. SACLA has been mainly used for material physics and biology, but our research group also applies it to elementary particle physics.
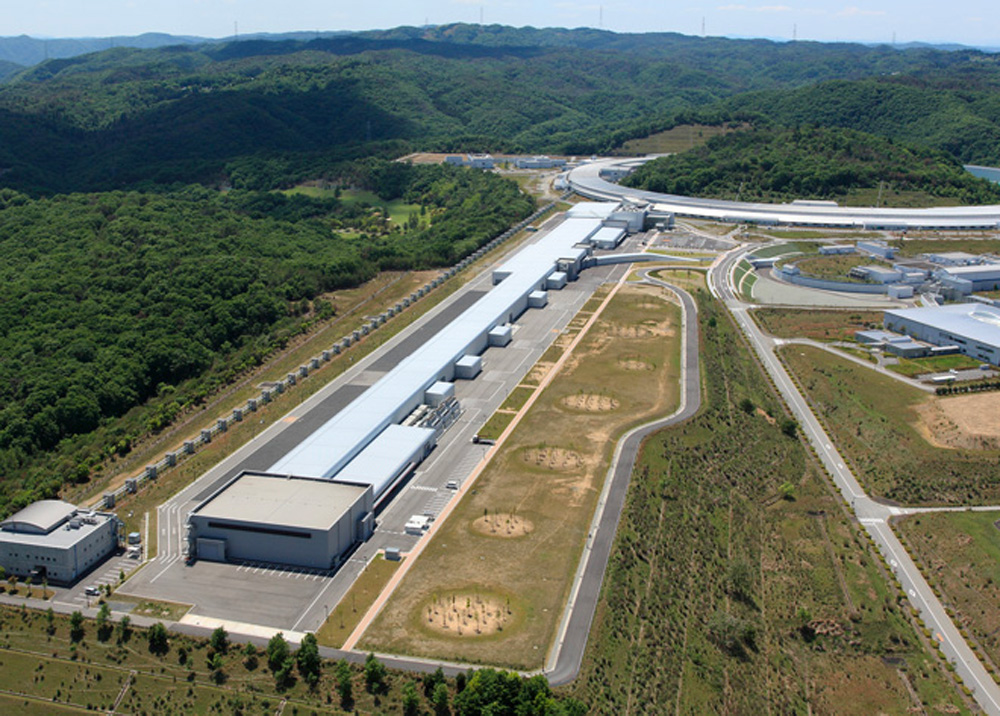