MEG Experiment
Muons,
the key to new physics
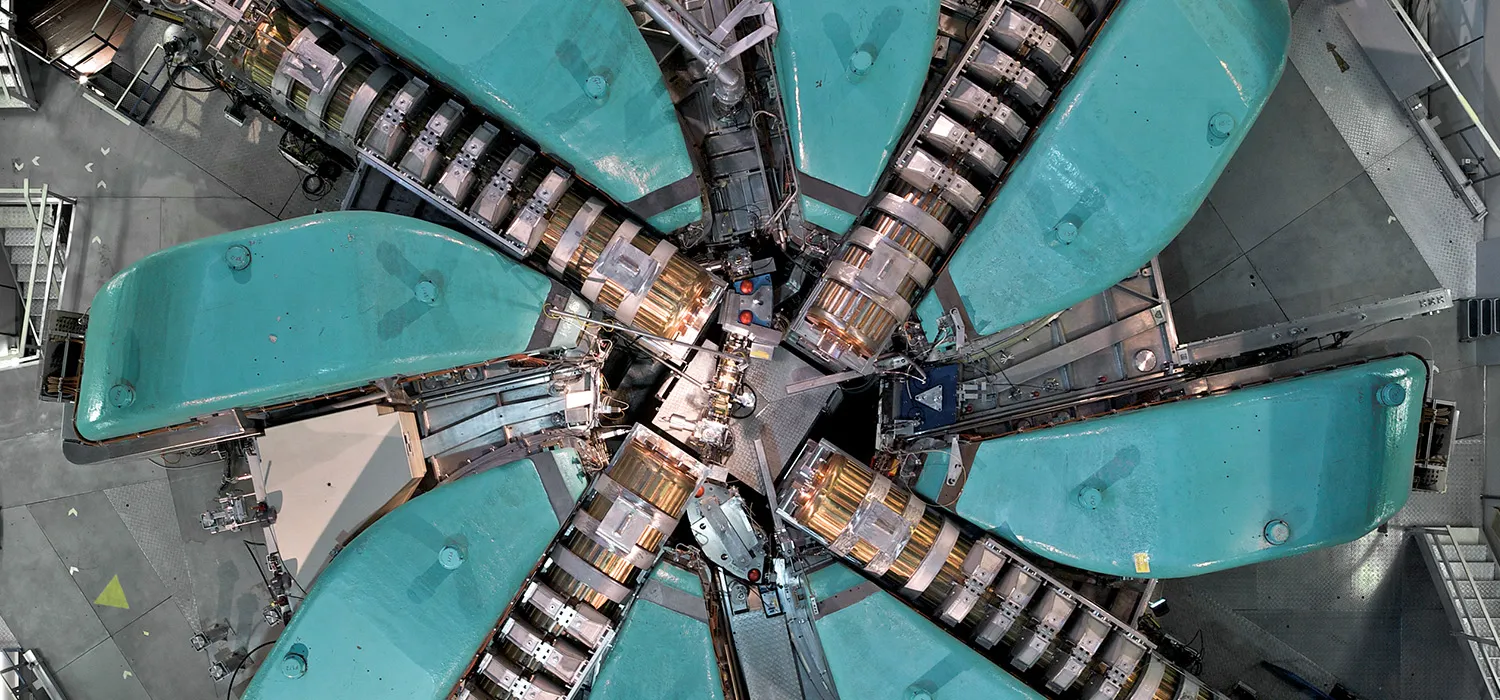
Overview
The MEG experiment is an experimental project that seeks to observe the "Mu to E Gamma (μ→eγ) decay" phenomenon in which a muon breaks down into an electron while emitting a gamma ray. The muon is an elementary particle similar to the electron classified as charged leptons. It has almost the same properties as the electron, but its mass is 200-fold mass.
The μ→eγ decay is forbidden in the Standard Model of elementary particle physics, but is predicted to occur in supersymmetric grand unified theories, which comprehensively explain the Universe and all the forces acting on the elementary particles, with an extremely low probability of once every several hundreds of billions to once every ten trillion muon decays. Discovering it would lead to prove the supersymmetric grand unified theories, while failing to observe it in the experiment would require revising them. In either case, this experiment is the key to establishing new paradigms of elementary particle physics.
The MEG experiment is an international joint research project initiated by the ICEPP researchers. Researchers from Italy, Switzerland, the U.S., and Russia who recognized the importance of the experiment joined the project and roughly 70 researchers have been conducting the project research since 2008 based in the Paul Scherrer Institute (PSI).
To observe the extremely rare μ→eγ decay, one firstly needs a large number of muons. This is made possible by PSI's proton cyclotron, the world's only facility capable of producing roughly 100 million muons per second. The Japanese research team has proposed and developed the major detector components, such as the COBRA spectrometer to measure positrons (positively charged electrons, e+) and the liquid xenon detector to detect gamma rays, as well as leads the whole project.
The experiment, which began in 2008, found that μ→eγ decay does not occur even once in every 2.4 trillion decays. This result set a very stringent constraint on theories that go beyond the Standard Model, such as the supersymmetric grand unified theories. Testing new physics theories further at higher levels of precision is imperative. The MEG II experiment will increase the experimental sensitivity by an order of magnitude and verify if μ→eγ decay occurs with a frequency of once every tens of trillions of decays. Start-up of the new experiment has been in progress since 2022.
PSI has future plans to create up to 10 billion muons per second, and has already begun considering detailed research experiments to perform following the discoveries that MEG II is expected to make.
The results of the MEG experiment have opened the doors for a new field of elementary particle physics - the charged lepton flavour physics. In this field, muons are used to verify new theories of elementary particle physics. Three other large-scale experiments are currently in progress in Japan, Europe, and the U.S. (the Mu2e, Mu3e, and COMET experiments). ICEPP holds international workshops that bring together the members involved in these experiments and is taking on the challenge of verifying supersymmetric theories through a global framework.
The mysteries of elementary particle generations
In the early 1970s, only three types, or flavours, of quarks had been discovered. Doctors Makoto Kobayashi and Toshihide Maskawa predicted six flavours of quarks that could be categorized into three generations.[B2] All of the quarks were later discovered, and experiments such as the Belle experiment at KEK confirmed that quark flavour transition between generations occurs just as predicted by the Kobayashi?Maskawa theory. In 2008, they received the Nobel Prize in Physics.
It was later discovered that neutrinos also undergo flavour transition. This is an area in which UTokyo researchers made significant contributions. In 1987, in the Kamiokande experiment, Dr. Masatoshi Koshiba, the founder of ICEPP, was the first to observe neutrinos from a supernova explosion (for which he received the Nobel Prize in Physics in 2002). In 1998, using Super-Kamiokande, a successor of Kamiokande with greatly improved observational capabilities, Dr. Takaaki Kajita found solid evidence of neutrino oscillation (for which he received the Nobel Prize in Physics in 2015).
The MEG experiment seeks evidence that charged leptons also undergo flavour transition. Supersymmetric grand unified theories predict that the transition will occur between muons and electrons, and the eyes of physicists around the world are focused on the results of the experiment.
Quarks and neutrinos have been confirmed to undergo flavour transitions. The MEG experiment aims to verify supersymmetric grand unified theories, which predict that charged leptons will also undergo flavour transitions (a violation of charged lepton flavour conservation), although this phenomenon will be very rare (roughly once every several tens of trillions of interactions).
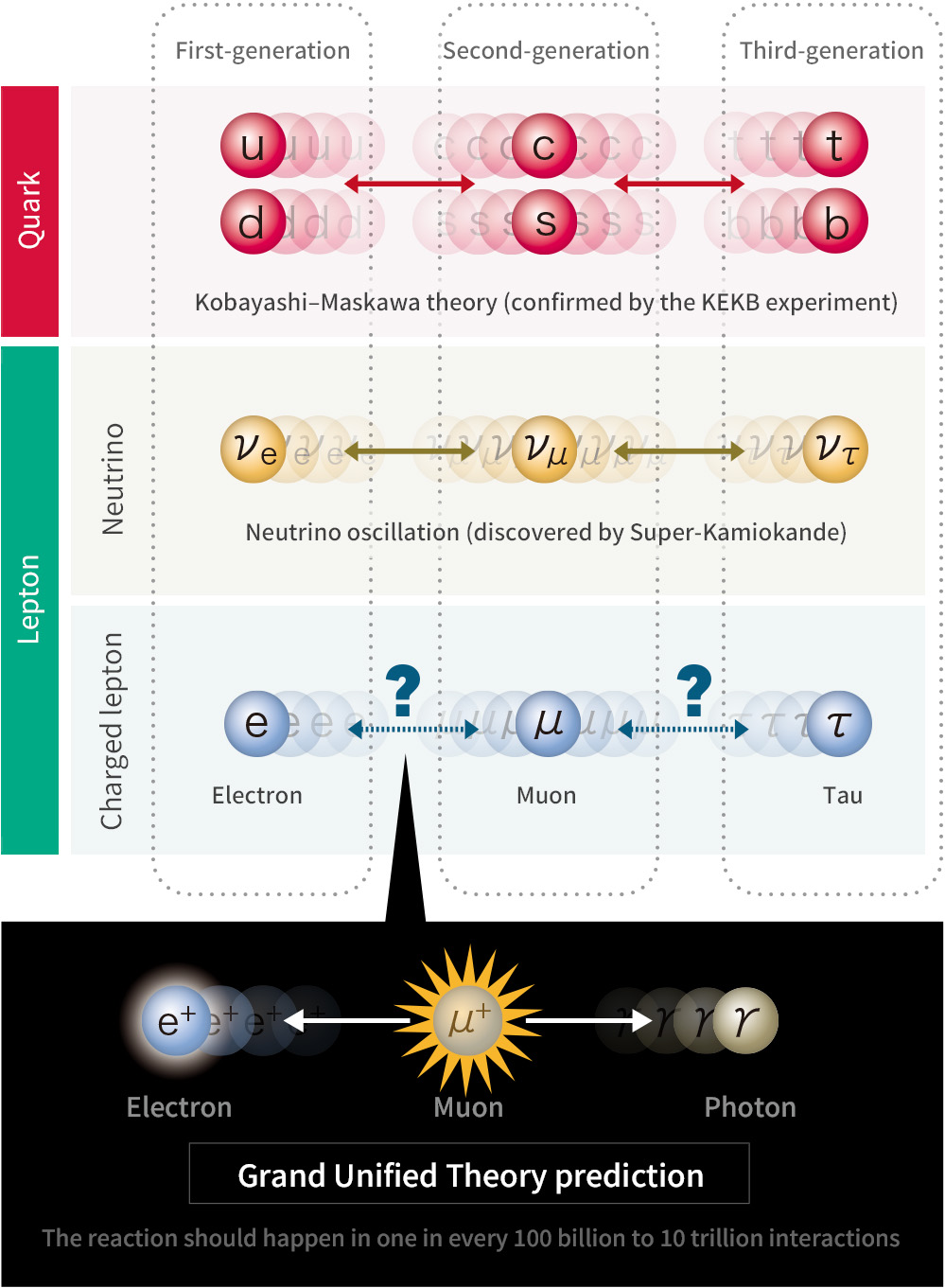